Imagine you’re trying to build the perfect racing car, but no one has ever built one before. There’s no single blueprint to follow. Some engineers might use a gas engine. Others might go for an electric. And yet others might try something entirely different, like a jet engine.
That’s the current state of quantum computers. There’s no definitive “best way” to build a quantum computer yet. Instead, about a dozen active research paths are vying for attention. Each approach has its own advantages and disadvantages.
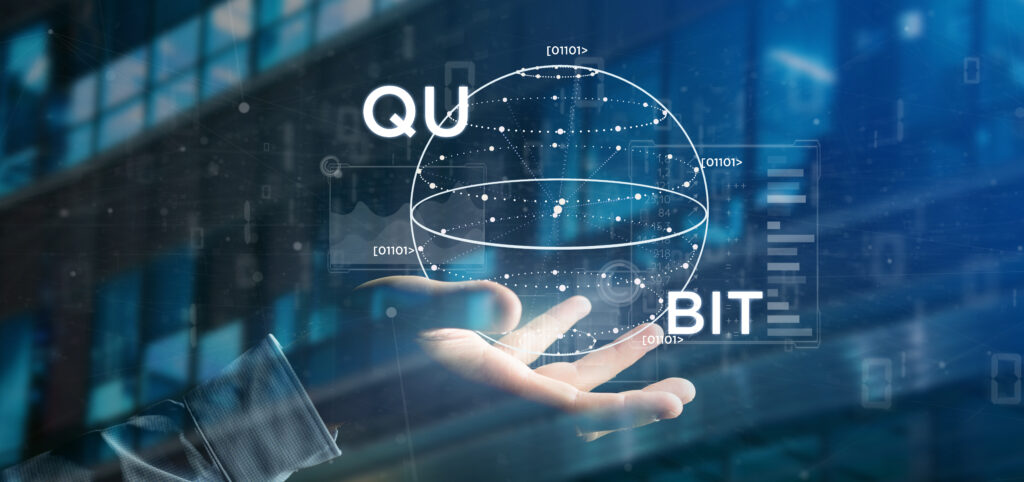
Key Terms Explained in Plain English
First, let’s define a few key terms. It’s impossible to explain the differences between types of quantum computers without referring to these concepts.
Qubits (quantum bits) – the basic units of information in a quantum computer.
In classical computers (like a smartphone or laptop), information is stored in binary “bits.” Bits can be in a state of either 0 or 1, and they’re the basic unit of information in computing.
Quantum computer scientists didn’t get very creative with naming conventions. In quantum computers, the basic unit of information is simply called a quantum bit, or qubit for short.
Qubits are the building blocks of quantum computers. They enable all the fun quantum mechanics like superposition or entanglement. These mechanics are integral to quantum computing, and the type of qubit dictates what’s possible and what’s not.
Coherence time – how long qubits can keep their quantum states?
In this field, qubit count is commonly treated as the be all, end all.
We’ve got a 400-qubit computer. Oh yeah? Well, we’re building a 500-qubit one… so take that!
It’s true that real-world use cases will need a large number of qubits. But qubit count alone doesn’t tell the full story. The coherence time of the qubit is also very important. This is basically how long a qubit can stay in a superposition state.
Some types of qubits might be harder to scale up in count, but they make up for it with longer coherence times.
Error rate – how often do mistakes occur?
Imagine you’re texting a friend, but sometimes your phone changes a word or two by mistake. Maybe it autocorrects “duck” to… well, something else. How often this happens is the “error rate” of your texting.
The error rate of quantum computers is important because they tend to be very sensitive. Even tiny temperature changes or a stray electromagnetic wave can mess with their calculations. Different types of quantum computers will have (sometimes very) different error rates.
Fault tolerance – is it accurate even when errors occur?
Picture a big choir. If one or two members sing off-key for a moment, the whole performance isn’t necessarily ruined. The other singers can help mask that mistake, and the overall song still sounds good. This choir has a “fault tolerance” for a few off-key notes.
A fault-tolerant quantum computer can complete its calculations correctly even when some qubits are “singing the wrong notes.” Fault tolerance is a prerequisite for quantum computing’s most impactful use cases, where millions of dollars (or even lives) could be at stake.
Scalability – can we add more qubits without breaking the computer?
Qubits are tricky to create, but they’re even trickier to scale. More computing power means more qubits, which means more opportunity for errors. Plus, increased interactions between qubits can also introduce errors.
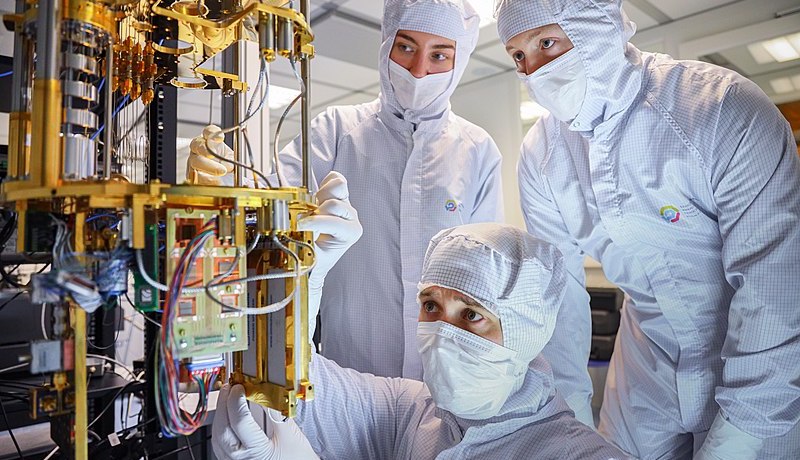
Types of Qubit Technologies
So, just how many types of quantum computers are there? In total, there are over a dozen active research paths. Let’s tour the most popular ones.
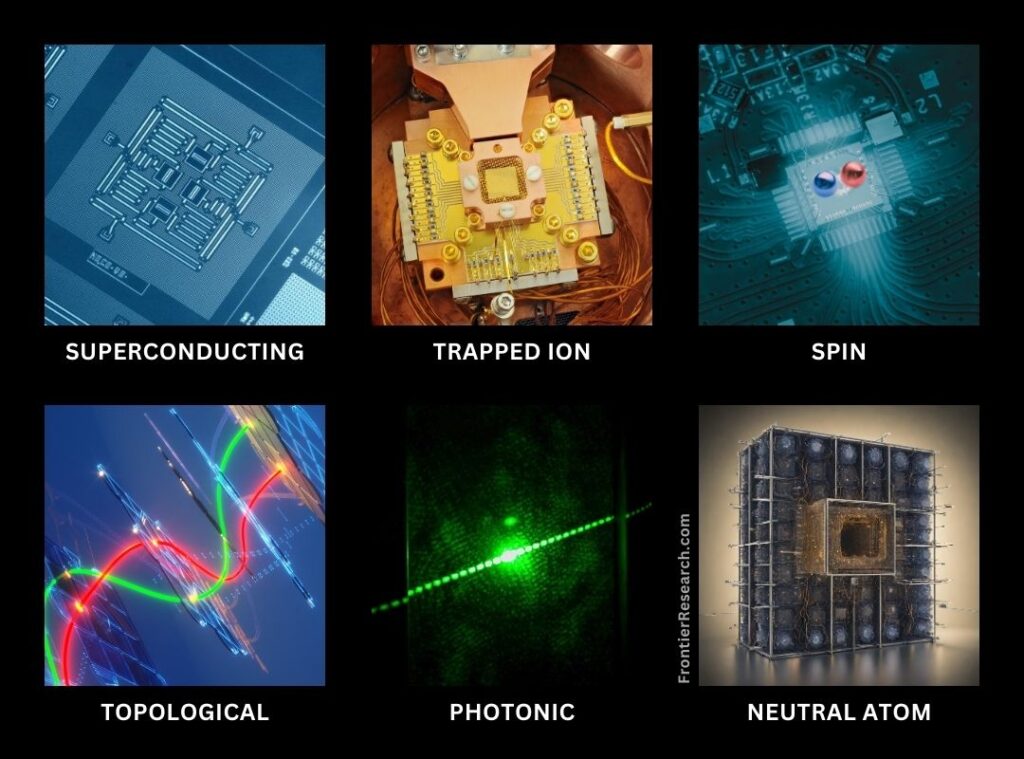
Superconducting Qubits
Superconducting qubits are tiny circuits made from materials that can conduct electricity perfectly. These quantum circuits need to be extremely cold to operate—colder than outer space.
Superconducting quantum computing is the most mature type of qubit. Big players like IBM and Google have been pushing this technology since the early 2000’s. They’ve also been featured in high-profile experiments, like Google’s claim to quantum supremacy in 2019.
The downside is that they need to be at cryogenic temperatures. They need to stay near absolute zero to keep their quantum states. As a result, they need a bulky, energy-consuming setup with huge dilution refrigerators.
Advantages | Disadvantages | Major Players |
Familiar technology Straightforward scaling playbook Easy to control Fast quantum gate operations | Sensitive to noise Short coherence times Need cryogenic temperatures | IBM Rigetti Computing Intel |
Trapped Ions
Take an atom and pull out one of its electrons. It now gets a positive charge, becoming an “ion.” In trapped ion qubits, we “trap” these ions using electromagnetic fields, like levitating tiny balls. We can then control these ions using lasers.
The main draw of trapped ions is that they’re resistant to noise. Because they’re suspended in a vacuum, they’re less likely to get errors from vibrations and such. They also have some of the longest coherence times of any type of qubit.
The downside is that scaling trapped ions is a technically complex problem. The more ions there are in a trap, the harder it is to control each ion without affecting the others.
Advantages | Disadvantages | Major Players |
Long coherence times Resistant to noise Precise, with low error rates | Hard to scale with precision Slower quantum logic gates More limited use cases Complex to build and manage | IonQ Honeywell Quantinuum Alpine Quantum Technologies |
Spin Qubits
Every electron has a property called “spin.” Think of it like the electron having its own little compass needle that can point either up or down. In spin qubits, we use this compass needle direction as a way to store quantum information.
One advantage of spin qubits is that they’re tiny, which means you can fit a lot of them in a small space. They can also be made with silicon, which we’re already very familiar with. Some types of spin qubits can even work at room temperature, without an expensive cooling system.
The downside is that spin qubits are harder to work with. First, getting spin qubits to interact with each other is tough. This limits the types of calculations they can do. It’s also harder to read out the information stored in a spin qubit.
Advantages | Disadvantages | Major Players |
Tiny; can be densely packed Long coherence times Can be made with silicon Some can operate at room temp | “Spin” is tricky to control Limited qubit connectivity Difficult to read out | Intel Silicon Quantum Computing Quantum Motion Toshiba |
Side Note: Quantum Dots
Quantum dots get a special mention here because you’ll see this term pop up. They’re related to spin qubits, but they’re not exactly the same thing. Think of quantum dots as tiny containers that can fit one electron at a time.
Spin is a popular choice for dot-based qubits, but charge qubits are also possible. Here’s an analogy to help differentiate. Imagine a room (the quantum dot) where a dancer (the electron) is either spinning (spin qubit) or simply stepping in and out of the room (charge qubit).
Topological Qubits
Imagine a knot. If you jostle or shake a well-tied knot, it doesn’t just come undone, right? It remains a knot. Topological qubits are based on that idea.
Instead of storing information in a particular property of a particle (like its spin or charge), they store it in the “knots” or twists of their quantum state. These knots are less likely to “untie” from minor disturbances.
Topological computers have the potential to be much more error-resistant than other types of quantum computers. However, topological qubits are still mostly theoretical and in the early stages of research. We’ve got ideas on paper, but making them real is a challenge.
Advantages | Disadvantages | Major Players |
Naturally more error resistant Longer coherence times Scalable | Require exotic materials to make Still largely theoretical | Microsoft |
Photonic Qubits
Photons are just particles of light. So, instead of relying on matter (like electrons in a superconductor or ions in a trap), we’re using beams or pulses of light.
Photons are useful because they don’t really interact with the environment much. That means they’re immune to many common forms of noise. They can also travel long distances at light. This makes them very attractive for quantum communication.
But photons are hard to control. It’s not easy making sure a single photon goes where you want and does what you want. The setup can also be bulky—think tables filled with mirrors, lasers, and beam splitters.
Advantages | Disadvantages | Major Players |
Resistant to environment noise Light speed travel Ideal for quantum communication Can operate at room temp | Hard to detect and control Bulky equipment required Limited photon connectivity Scaling challenges | Xanadu Quantum Technologies ORCA Computing PsiQuantum BosonQ |
Neutral Atom Qubits
Neutral atom qubits are individual atoms that aren’t charged (they have the same number of electrons as protons). These atoms can then store information in different energy levels. Neutral atom qubits and trapped ions both use atomic systems, but they’re not the same thing.
For example, trapped ions interact more strongly because they are charged. This is both a pro (for certain operations) and a con (potential noise). Neutral atoms have weaker interactions, and can be packed more densely.
Advantages | Disadvantages | Major Players |
Potential for high qubit density Excels at parallel processing Very precise and stable | Interference between atoms Low interaction strength Limited use cases Complex to build and manage | QuEra Computing Atom Computing Pasqal ColdQuanta |
Other Types of Quantum Computers
The ones above are currently the most popular types of quantum computers. They have seen the most research and development in recent years. Other types include:
- NV (Nitrogen-Vacancy) Center Qubits. NV center qubits are defects in diamonds. It’s where a carbon atom is replaced by a nitrogen atom, leaving a vacant spot next to it.
- Rydberg Atom Qubits. These are atoms that are excited to a very high-energy state called a Rydberg state.
- Majorana Fermion Qubits. Majorana fermions are quite unique. They are their own antiparticles, meaning they can annihilate themselves. In quantum computing, we’re not using actual Majorana fermions, but “quasiparticles” that act like them.
- Atomic Ensemble Qubits. Atomic ensemble qubits don’t rely on a single atom. Instead, they spread out quantum information over an entire group.
- Linear Optics Qubits. These use properties of light, such as polarization, to store information. They’re affected more by noise than photonic qubits, but are easier to make.
- Nuclear Magnetic Resonance (NMR) Qubits. These use the nuclear spins in molecules. These were one of the first types of qubits, but are less popular today.
- Surface code qubits. This is a method for error correction. It involves arranging qubits on a two-dimensional grid. This grid of qubits is designed to detect and correct errors.