From the abacus to iPhones, each leap in computing power has changed society. Quantum computing will be no different, but like those early room-sized computers, the quantum machines of today are fragile and delicate. Let’s explore this technology born from the wildest theories of physics, understand its challenges, and why its potential is so immense. While stable, scalable quantum computers are still years away, this is absolutely something you should keep a close eye on – whether you’re an investor, tech-obsessed, or just plain curious.
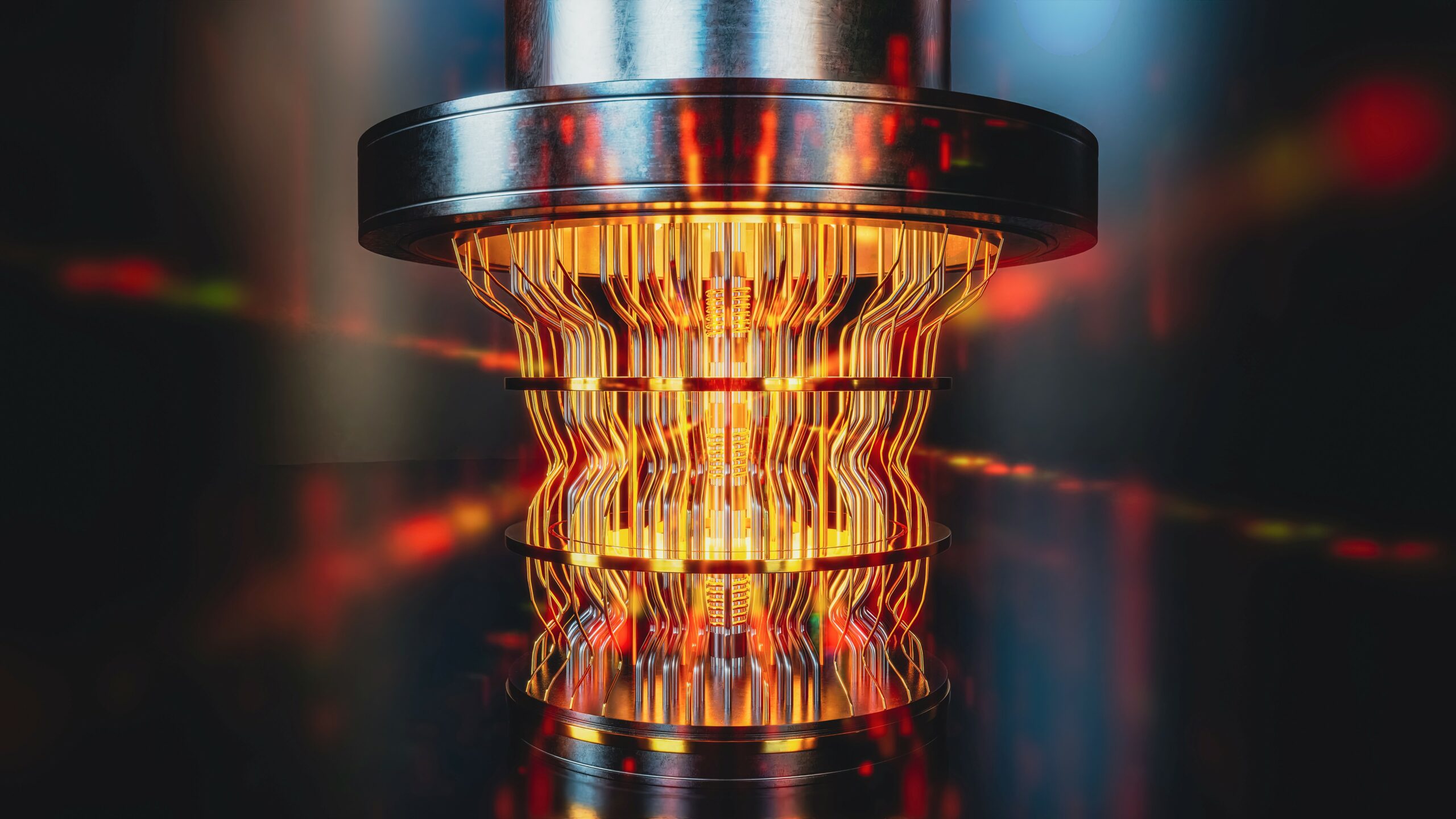
What is a Quantum Computer?
At their core, the computers we use today are digital machines built on “bits.” Bits are the smallest unit of information, represented as either a 0 or a 1. All the complex tasks our laptops and smartphones handle – from streaming videos to spreadsheets – are basically recorded as an ocean of simple 0’s and 1’s. Classical computers have changed the world, but they’re already reaching their limits when tackling problems of extreme complexity.
Bits vs. Qubits
Enter the quantum bit, or qubit for short. Unlike a classical bit forced into either “on” or “off,” a qubit can be both 0 and 1 simultaneously. It’s a mind-bending concept from quantum physics called “superposition.” Think of a coin flipped into the air. While it’s spinning, it’s both heads and tails at the same time. Only when it lands does it settle into a definite state.
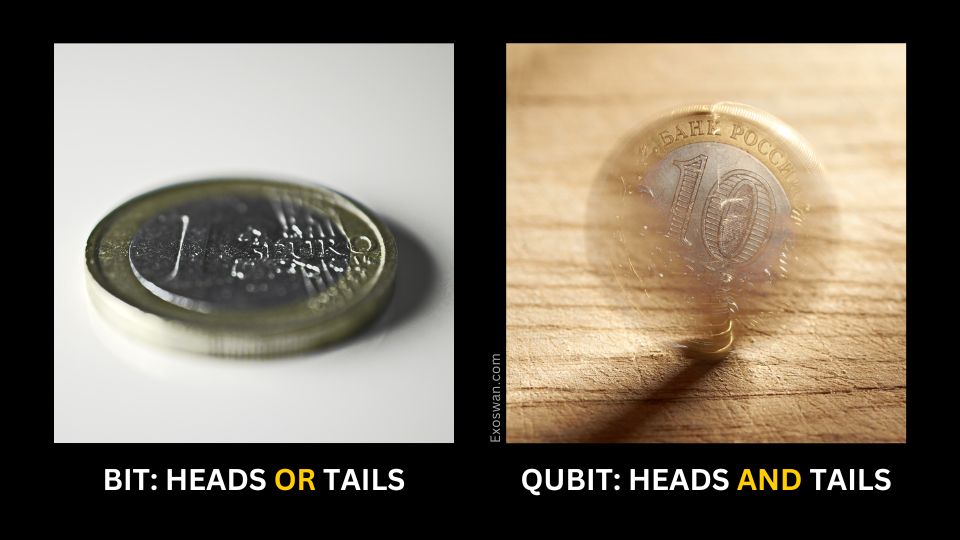
This is where things get interesting. Two qubits in superposition can represent four states at once (00, 01, 10, 11). Add a third qubit, and it jumps to eight states. The scaling is exponential. This is the type of boost that makes quantum computers so powerful – they explore multiple possibilities all at once. This fundamental difference is what opens the door to a whole new way of computing.
Bit State | Decimal Equivalent |
000 | 0 |
001 | 1 |
010 | 2 |
100 | 3 |
011 | 4 |
101 | 5 |
110 | 6 |
111 | 7 |
Superposition and Quantum Entanglement
Quantum computers rely on two strange but powerful phenomena from quantum physics: superposition and entanglement.
- Superposition: Remember our spinning coin analogy? Superposition is the qubit’s ability to embody multiple states (0 and 1) at once until it’s measured, like the coin being both heads and tails until it lands. This allows qubits to process a huge range of possibilities simultaneously.
- Entanglement: Entanglement is a strange quantum phenomenon where two qubits become inextricably linked. If one qubit is measured as 0, the other instantly “knows” and collapses to 1, even if they’re far apart. In the coin analogy, imagine two coins that get flipped into the air. These two coins could be separated by thousands of miles, but the moment one lands as “heads,” the other lands as “tails.” This type of link unlocks further computational power and enables quantum communications.
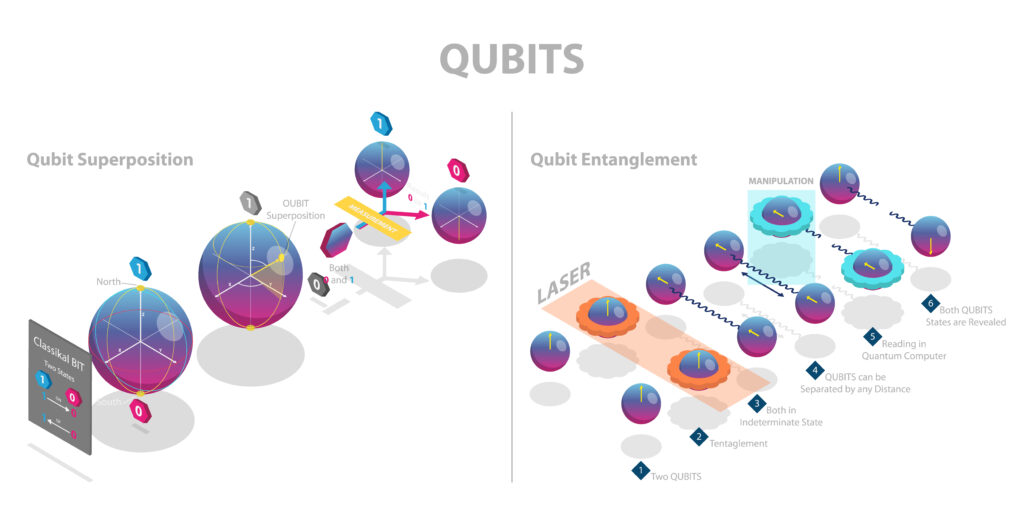
The Power of Quantum Parallelism
The magic of quantum computing arises from its ability to break free from the step-by-step limitations of classical computing. A classical computer tackling a problem is like a determined explorer in a maze, checking one path at a time, potentially backtracking and hitting dead ends.
On the other hand, the “fuzziness” of qubits allows a quantum computer to do things differently. Thanks to superposition, a quantum computer can essentially explore many paths of the maze all at once. This is called quantum parallelism, and it’s the source of those headline-grabbing speed-ups.
Imagine a vast field of potential solutions. A classical computer painstakingly checks them one by one. We can speed this process up with engineering efforts, as we build increasingly powerful chips that process information faster. But there’s still an inherent limitation due to the way the computer works at a fundamental level.
On the other hand, a quantum computer can cast a ‘quantum net’ over the entire field. This significantly increases the chance of finding the optimal solution, or even solutions we didn’t know existed. This power shines brightest in scenarios where sheer brute-force calculation fails, such as deciphering complex encryption or simulating new medicines.
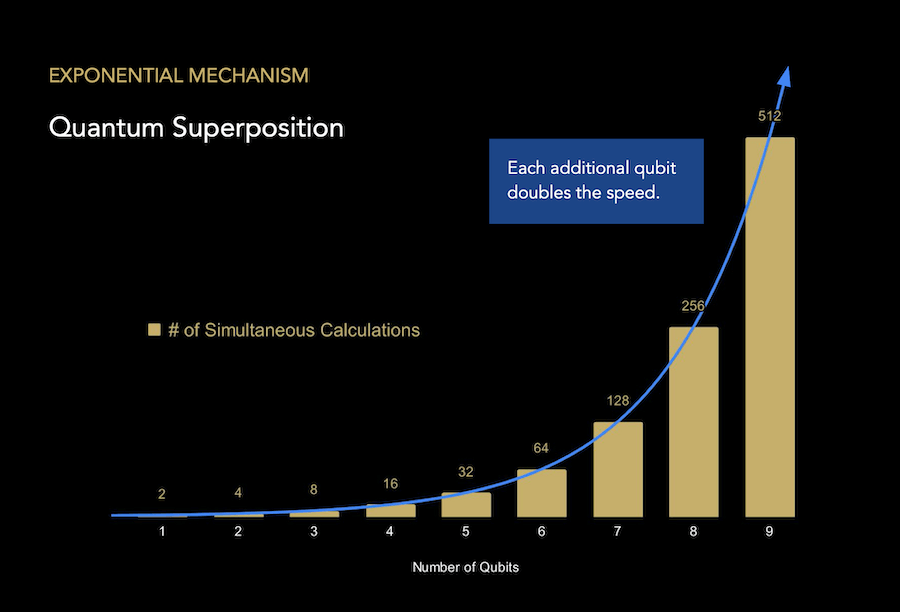
Reality Check: Quantum Computing is Not a Magic Bullet
Keep in mind though: Quantum computers aren’t magic bullets. They excel at specific types of problems, but won’t replace your laptop. Imagine them as highly specialized tools that solve problems deemed too complex for traditional computers. While most of your daily computing tasks will remain a better fit for a standard computer.
Also, know that today’s quantum computers are incredibly delicate. Think of them less as computers and more like finicky lab experiments. They need ultra-cold environments and are susceptible to errors. Building and maintaining a large, reliable quantum computer is a massive (and expensive!) scientific challenge.
This field is still young, like the clunky room-sized computers of the past. We’re in the era of noisy, error-prone machines. While powerful, their potential rests on whether scientists and engineers can scale the technology and reduce errors to make them truly practical.
Potential Impact on Industries and Society
Should quantum computing reach its full potential, we’ll absolutely see widespread disruption. However, different industries will be impacted at different speeds.
Cryptography and Cybersecurity
Quantum computers pose a grave threat to existing cryptography due to quantum algorithms like Shor’s algorithm. This algorithm allows them to break encryption that would take centuries for classical computers to crack, potentially exposing everything from private messages to military secrets. This vulnerability, however, serves as a driving force to develop post-quantum cryptography – new encryption methods designed to be secure even against quantum attacks. This creates a cat-and-mouse game, propelling us towards a future of quantum secure communication (QSC).
The key challenge lies in being proactive. Since encrypted data can be stolen now and decrypted later when quantum computers mature, we need to start transitioning to quantum-resistant cryptography urgently. While the threat is real, quantum computing’s impact on cryptography is two-sided – forcing us to not only break our current security protocols, but also to develop even stronger replacements.
Drug Discovery and Materials Science
At the heart of drug discovery and materials design lies the need to understand and predict the behavior of complex molecules. Classical computers hit a wall here – the calculations simply become far too complex. It’s like trying to predict the outcome of a million coin flips happening at once. This leaves approximations and guesswork as the only tools, slowing down the development process.
Quantum computers change this game by being ‘naturally suited’ to simulating quantum systems like molecules. Their ability to hold many states simultaneously mirrors how electrons behave in complex molecules. This could allow for precise prediction of a molecule’s properties before it’s even synthesized! This translates to faster identification of drug candidates for complex diseases and the ability to virtually design materials with specific properties, such as ultra-strong yet lightweight alloys for aerospace or revolutionary new battery materials.
Optimization Problems in Logistics and Finance
Many real-world problems in logistics and finance boil down to finding the best solution from a mind-boggling number of possibilities. Think about a fleet manager trying to find the most efficient delivery routes for a thousand trucks factoring in traffic and fuel costs, or an investor trying to build a portfolio with the optimal balance of risk and reward across thousands of assets. Classical computers often get stuck in local optima – decent solutions, but not the globally best ones.
Quantum computers, with their ability to explore many possibilities simultaneously, could provide the key to unlocking these true ‘best’ solutions. Algorithms like Quantum Approximate Optimization have the potential to cut through the combinatorial complexity that plagues classical computers. Imagine near-instant re-routing of a delivery fleet in real-time as traffic snarl-ups emerge, or portfolio models that can dynamically adjust holdings to seize opportunities invisible to traditional analysis. This ability to find more optimal solutions translates to lower costs, smarter resource allocation, and significant competitive advantages in fast-moving markets.
Artificial Intelligence and Machine Learning
AI and machine learning thrive on analyzing massive datasets to uncover patterns and make predictions. However, classical computers can struggle with the sheer size and complexity of some datasets, particularly for tasks like image recognition or natural language processing. Quantum computers could augment AI in several ways. Imagine quantum machine learning algorithms that uncover correlations and patterns that would completely escape classical systems, potentially leading to leaps in AI capabilities.
Another exciting area is in accelerating the training of complex AI models. This training process can be computationally very expensive. Quantum optimization could drastically slash the time this process takes.
Imagine a self-driving car that instantly reacts to unpredictable traffic, not just what it was programmed for. Or a medical system spotting patterns in patient data a human doctor might miss. Quantum computing could make these kinds of AI breakthroughs a reality. We’re still in the early days, but this combo has the potential to unlock truly intelligent systems.
The Current State of Quantum Computing
Right now, we’re in a heated race to push quantum capabilities forward, with a mix of tech giants, government initiatives, and smaller startups battling it out. This race is driven by the recognition of the immense potential, but also the extraordinary difficulty of the task. The main focus currently is on:
- Increasing Qubit Count: Today’s quantum computers have relatively few qubits. Researchers are pushing to increase this while maintaining qubit quality.
- Error Correction: Qubits are error-prone. Finding reliable ways to correct these errors is essential for scaling up computations.
- Developing “NISQ” Algorithms: These are algorithms designed for today’s “noisy” quantum computers. Scientists are trying to find uses for these imperfect machines even before we reach large-scale reliability.
NISQ: Noisy Intermediate-Scale Quantum
The term NISQ, or “Noisy Intermediate-Scale Quantum,” describes the current state of quantum technology. Here’s what it signifies:
- Noisy: Today’s quantum computers are prone to errors. Environmental factors and imperfections in the hardware contribute to a high degree of “noise” that disrupts computations.
- Intermediate-Scale: We’re in the era of machines with a moderate number of qubits – think tens or hundreds, rather than the millions likely needed for full-blown error correction and applications like breaking modern encryption.
- The Imperfect Advantage: While far from the ideal of a large-scale, fault-tolerant quantum computer, NISQ devices offer intriguing potential. Researchers are developing algorithms that cleverly work around the limitations of these machines, aiming to find areas where they might still outperform classical computers.
- Potential Applications: NISQ algorithms often focus on hybrid approaches, where the quantum computer tackles a specific computationally hard part of a problem, while a classical computer handles the rest. Early applications could emerge in areas like materials simulation, optimization problems in finance, or aspects of drug discovery.
Think of NISQ as a stepping stone. It’s about making the most of the imperfect machines we have today while we continue striving for the more powerful quantum computers of the future.
Major Players in the Field
From tech giants to scrappy startups, it’s a horse race of different approaches to quantum approaches. This mix of players highlights the collaborative nature of the field, with competition driving rapid progress.
Tech Leaders
IBM, Google, Microsoft, and Amazon are leading the quantum charge. In 2019, Google made headlines with their claim of “quantum supremacy,” where their Sycamore processor crunched a problem that would choke a traditional supercomputer. While the calculation itself was niche, it was a symbolic milestone. IBM continues to set records for qubit count, like their 433-qubit Osprey chip. Meanwhile, Microsoft and Amazon are focused on the behind-the-scenes stuff: the cloud software that’ll one day let businesses tap into quantum power.
Government Initiatives
Quantum computing isn’t just a tech race – national security is on the line. In the US, the National Quantum Initiative Act poured serious money into quantum technologies. This money established research centers across the country and aims to train a quantum-ready workforce. China is also heavily invested in quantum technology, with major research hubs and aggressive goals. The EU has its own Quantum Flagship program, funneling billions into basic research and developing commercial applications.
Startups and University Labs
Beyond the tech giants, “pure-play” startups like IonQ, Rigetti Computing, and D-Wave Systems are focused on building better quantum hardware. IonQ is betting on trapped-ion qubits, while Rigetti on superconducting circuits. D-Wave Systems has taken a different path altogether with their specialized “quantum annealing” machines. University labs remain the foundation of quantum progress. Institutions like MIT, Caltech, and the University of Waterloo are driving breakthroughs in error correction, algorithm development, and fundamental quantum physics. These advancements often pave the way for the technologies used by commercial players.
Building a Quantum Computer: Challenges & Limitations
The holy grail of quantum computing lies in achieving systems that both boast vast processing power and can reliably handle errors. To reach this point, significant milestones remain:
Decoherence: The Fragile Nature of Qubits
Qubits, the heart of quantum computers, are incredibly delicate. The slightest interaction with the environment (think heat, stray electromagnetic fields) can cause them to lose their superposition state. This causes quantum information to ‘leak’ away, disrupting calculations. Maintaining coherence for long enough to perform meaningful computations is a huge engineering challenge, requiring near-perfect isolation of the quantum system.
Error Correction: Combating Quantum Noise
Qubits aren’t just fragile, they’re also prone to errors during operations. Developing reliable error correction techniques is a major bottleneck. Some error correction strategies involve using many physical qubits to represent a single, more reliable “logical qubit,” adding overhead and complexity.
Scaling Up: The Qubit Hurdle
Today’s most advanced quantum computers have only a few hundred qubits. Useful applications in areas like breaking modern encryption may require millions of high-quality qubits. Scaling up while maintaining coherence and low error rates is incredibly difficult. It’s not just about making more qubits, but making better qubits.
The Algorithm Gap
While quantum hardware gets attention, quantum software development is equally important. We need new algorithms designed to harness the unique way quantum computers work. Current algorithms often struggle to offer a clear speed advantage over classical computing for real-world problems.
Hype vs. Reality
Media often focuses on potential breakthroughs, but the journey to practical quantum advantage is long. While exciting, the field is still young. Early quantum devices are noisy, error-prone, and best suited for research rather than everyday business applications.
The Future of Quantum Computing
The quantum race is in full swing. It’s hard to determine the true starting line, but Google’s 2019 milestone of “quantum supremacy” was certainly the inflection point.
Quantum Supremacy and Its Implications
The concept of quantum supremacy marks a theoretical turning point: the moment a quantum computer definitively outperforms any classical computer, however niche the task. Google’s 2019 claim ignited this conversation. Their quantum processor, Sycamore, performed a calculation in mere minutes that they argued would take the most powerful classical supercomputers thousands of years. While controversial, with critics debating the task’s practicality, it was an undeniable landmark.
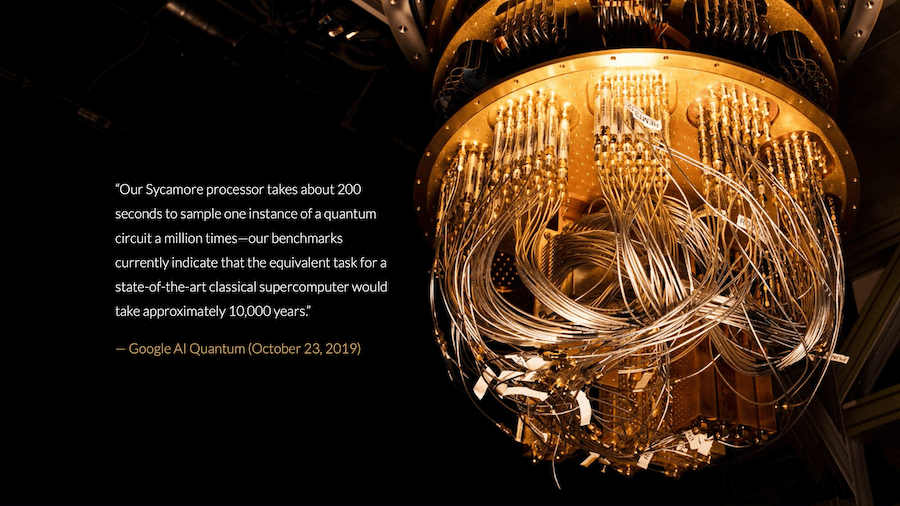
Google’s achievement serves as a proof of concept: quantum computers aren’t mere theoretical curiosities. They have the potential to surpass even our most powerful classical systems. This milestone fuels a global race, spurred by both investment and competition, as labs and tech giants chase the next breakthrough in quantum power.
However, managing expectations is key. Quantum supremacy on specific tasks doesn’t immediately translate to industry-transforming machines. Current demonstrations are exciting, but shouldn’t be mistaken for quantum computers suddenly being poised to disrupt entire industries overnight. Think of quantum supremacy as a symbolic marker inspiring the push towards the true goal of widespread quantum advantage.
The Road to Scalable, Fault-Tolerant Quantum Computers
Right now, the “best” approach to building a stable, scalable quantum computer is still unknown. The path to building powerful, reliable quantum computers hinges on several key areas where different approaches are vying for dominance.
Qubit Architecture
This is the heart of the debate. The quest to build reliable quantum computers hinges on the choice of qubit architecture. Leading contenders like superconducting qubits (relying on electrical currents in ultra-cold materials) are well-established but complex to engineer. Trapped ion qubits (using lasers to manipulate individual ions) offer long coherence times but struggle with scaling to large numbers. The holy grail remains theoretical topological qubits, which promise inherent error resistance due to their unique properties, but remain a major scientific challenge to physically realize.
Significant research is ongoing to improve existing qubit methods and explore entirely new avenues. This fierce competition is a positive force, accelerating progress towards the ultimate goal – a robust, scalable quantum computer.
Error Correction Techniques
All approaches grapple with errors, which are the enemy of reliable quantum computation. Researchers are pursuing various error correction techniques. Quantum error correction codes cleverly encode one logical qubit across multiple physical ones to allow for error detection and correction, but this adds complexity and overhead. Another avenue is fault-tolerant hardware design, striving to create qubits with built-in resistance to errors, potentially reducing the need for complex correction schemes.
Scalability and Interconnectivity
Building a quantum computer isn’t just about creating better individual qubits; it’s about scaling up and connecting them. Think of it as the difference between a single powerful light bulb and a vast, interconnected power grid.
Current quantum computers have relatively few qubits, and increasing this count while maintaining quality is essential for tackling meaningful problems. This requires advances in fabrication techniques, as well as new ways to efficiently control and manipulate the growing number of qubits.
Additionally, as qubit numbers rise, we need ways to entangle and coordinate operations between them. This involves developing “quantum communication channels” or ways to shuttle quantum information around a complex system. It’s analogous to building a vast network of roads after we’ve mastered how to build powerful cars.
Realistic Timelines for Practical Quantum Computing Applications
Predicting timelines in a rapidly evolving field is like nailing Jell-O to a wall. It’s notoriously difficult, but understanding the general trajectory can help us have realistic expectations:
- Near-Term (2-5 years): We’re already seeing companies like Volkswagen and Merck dabbling with NISQ devices for specific tasks. Expect continued progress with these machines and more experimental use cases emerging in areas like materials science and early drug discovery. Limited demonstrations of quantum advantage in specialized applications might become more common.
- Medium-Term (5-15 years): Breakthroughs in error correction or qubit technology could accelerate timelines. We could see the development of more robust quantum systems capable of tackling problems that are currently intractable for classical computers, though these might still be niche applications. Industries like pharmaceuticals or finance could begin to explore real-world use cases, but classical computers are likely to remain crucial partners.
- Long-Term (15+ years): The ultimate goal of fault-tolerant machines capable of breaking modern encryption remains a significant challenge. While this time frame might still hold, recent advancements suggest it could be achieved sooner with continued research and funding. Disruptive discoveries can always alter timelines, so this remains an estimate.
It’s important to remember that widespread adoption of quantum computing will likely be a gradual process. Early applications will co-exist with classical computing, with each playing to their strengths. The true impact will unfold as both hardware and software mature, making quantum computing a more accessible and user-friendly technology.
Investing in the Future
Big tech, big governments, and startups alike are betting big on quantum computing. After all, the potential rewards for cracking medicine’s toughest problems or revolutionizing finance are immense. But keep in mind that this is bleeding-edge technology – some of today’s players won’t exist in a few years.
At Exoswan Insights, we have a proven track record in identifying exponential technologies early, with some of our past picks surpassing 100X returns. To navigate this tricky landscape and stay ahead of the quantum revolution, join our newsletter today.