In a not-too-distant future, a hospital technician presses a button and create a brand-new organ for a patient. This organ is customized right down to the tiniest cell, all but eliminating the risk of rejection. “Waitlists” for transplants are but a relic of the past, and even lost limbs or teeth can be replaced. This sounds like a scene straight out of Blade Runner or Star Trek — but it’s closer to becoming a reality than you might think. This dream of medical miracle is the real-world endgame of the emerging technology called 3D bioprinting.
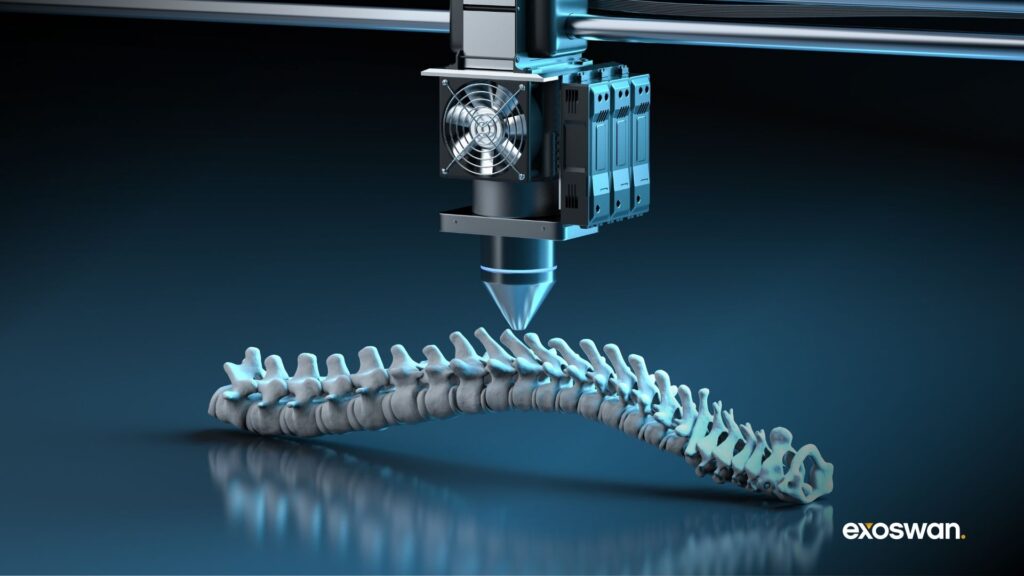
What is 3D Bioprinting?
3D bioprinting is the art and science of manufacturing living tissues and organs, layer by meticulous layer. If you’ve heard of 3D printing, you’ve likely seen those small desktop printers that spit out plastic trinkets, parts, or prototypes—sometimes useful, often frivolous. Bioprinting takes this concept and turbocharges it by adding living cells, biological materials, and other vital substances to the mix. Think of it as a 3D printer that instead of printing with plastic, prints with “bioink”—a mix of living cells and the supportive goo that holds them in place.
The difference between printing a chess piece and printing a functioning human organ may seem vast—and, to be honest, it is. But the fundamental principle remains the same: add material layer by layer until you get the desired three-dimensional structure. This time, however, the material you’re adding are living cells.
What’s the Difference Between 3D Printing and Bioprinting?
For one, there’s a lot more at stake. If you mess up while printing a little plastic Yoda for your desk, no problem—you can scrap it and start again. But when you’re building a patch for a human heart or a section of skin for a burn victim, you can’t afford the same luxury. 3D printing works with metals, plastics, ceramics—you name it. But bioprinting uses bioinks composed of living cells, nutrients, and a matrix to support cell growth.
In traditional 3D printing, the goal is precision. Precision in shape, weight, and aesthetic quality. With bioprinting, there’s a different standard—precision in life. Cells have to align correctly, survive the printing process, and continue to grow in an organized, functional manner afterward. In a sense, 3D printing is about perfection of form, while bioprinting is about perfection of function.
Recent Advances in 3D Bioprinting
In recent years, 3D bioprinting has moved from a dazzling proof-of-concept to practical application. Researchers have managed to create bioprinted heart valves, skin grafts, and even vascular channels—the latter being key for creating complex organs that need a blood supply. For example, scientists at Harvard’s Wyss Institute developed a bioprinted cardiac patch capable of contracting and mimicking real heart tissue. Researchers at Wake Forest Institute for Regenerative Medicine have also successfully printed ear, bone, and muscle structures that, once implanted in animals, matured into functional tissues.
This isn’t just about pushing the boundary of what is possible; it’s about saving lives and dramatically changing the medical field. Companies like Organovo, CELLINK, and 3D Systems have made strides in producing tissue that’s functional enough to test drugs on—a critical development that could help make drug testing both faster and safer. Organovo, for example, has developed liver and kidney tissue models used for drug toxicity testing. CELLINK, meanwhile, has developed specialized bioinks for a variety of research applications.
In 2019, Israeli scientists managed to print a small heart—not one ready for implantation, but one that serves as a starting point for something much larger. The heart was printed with human cells and included blood vessels, ventricles, and chambers, showcasing the early capability to create functional cardiac structures. Additionally, researchers at Utrecht University in the Netherlands have made significant progress in bioprinting kidney tissues with functioning tubules, laying groundwork for future organ-level developments.
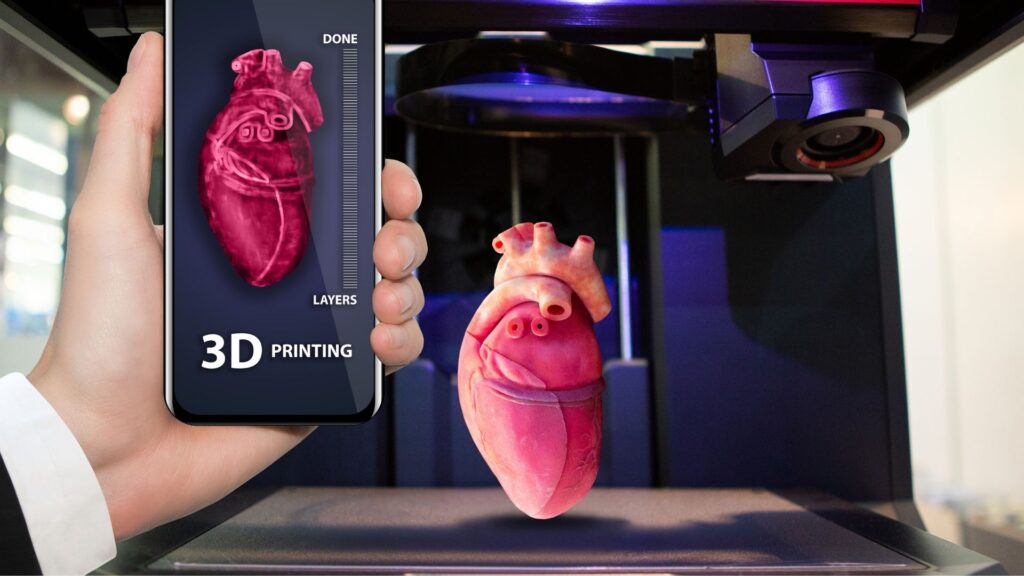
How Does 3D Bioprinting Work?
The magic of bioprinting happens at the intersection of biology, engineering, and robotics. Think of it as a very precise and delicate form of cake icing—only, instead of sugar, we’re talking about living cells, and instead of cake, the end product might just save a life. Let’s break down how this all works.
Bioinks
Bioinks are to bioprinters what plastic filaments are to standard 3D printers—the basic material. But, unlike plastic, bioinks must meet a couple of complex requirements. They need to provide structural support, maintain cell viability, and be biocompatible, meaning they won’t be rejected by the body. The bioink usually consists of a mix of cells and a hydrogel—something akin to Jell-O that provides a supportive matrix.
In a perfect world, every bioink would contain exactly the types of cells needed for a specific tissue—heart cells for a heart, skin cells for skin, etc. But we’re not quite there yet, so scientists often use stem cells, which can be coerced into becoming different cell types depending on what’s needed. This flexibility makes stem cells a kind of blank canvas—versatile and capable of filling many roles in the body.
Types of 3D Bioprinters
The types of 3D bioprinters vary based on the technique they use to deposit cells. The three most common types are inkjet, extrusion-based, and laser-assisted bioprinters. Each of these printers has its niche, and researchers often choose the type of printer based on the kind of tissue they need to create.
- Inkjet Bioprinters: These printers are the closest cousins to your average desktop printer. They “spray” droplets of bioink, similar to how an inkjet printer sprays ink onto paper. These are particularly useful for creating relatively simple structures, like sheets of skin tissue.
- Extrusion-Based Bioprinters: These work by squeezing out bioink through a nozzle, similar to how toothpaste is squeezed from a tube. This technique is better suited for printing larger, more complex tissues that require more layers.
- Laser-Assisted Bioprinters: This might sound like something out of a sci-fi movie, but it’s real and quite elegant. In this method, laser energy is used to focus on a bioink layer, which results in very fine, precise droplets of material being deposited. It’s extremely accurate and suitable for detailed, complex structures, but tends to be more expensive.
Printer Type | Inkjet | Extrusion-Based | Laser-Assisted |
---|---|---|---|
How It Works | Sprays droplets of bioink, similar to inkjet printing | Squeezes bioink through a nozzle like toothpaste | Uses laser energy to deposit bioink in precise droplets |
Pros | Low cost, relatively simple to operate | Suitable for larger, complex tissues; versatility | Extreme precision, suitable for complex structures |
Cons | Limited complexity; lower cell viability | Lower precision, risk of nozzle clogging | High cost, complex setup and operation |
Tissue Use | Skin sheets, simple tissues | Cartilage, bone, large patches of tissue | Vascular tissues, highly detailed organ sections |
Examples | Organovo, CELLINK | CELLINK, EnvisionTEC | Poietis, Advanced Solutions Life Sciences |
Advantages & Benefits of 3D Bioprinting
There’s something a little mind-bending about a printer that doesn’t make a toy or a gadget, but a living, breathing piece of flesh. There are many potential benefits to this technology, and we’re just beginning to scratch the surface.
Personalized Medicine
Commercialized 3D bioprinting could usher in an era where the waiting list for organ transplants is obsolete. Instead of crossing your fingers for a match from a dwindling donor pool, you could receive an organ printed specifically for you—tailored down to the cellular level to match your body’s biology.
Many patients die waiting for an organ. Even those fortunate enough to receive a transplant still face the lingering threat of rejection. Bioprinting promises to solve this by creating organs that are genetically identical to the patient’s own tissue, reducing the risk of rejection dramatically. Beyond organs, bioprinting could produce custom tissue patches for surgeries, tailored cartilage replacements, or a host of other personalized treatments.
More Accurate & Ethical Trials
Another major advantage is in pharmaceutical testing. Current drug trials often begin with animals, but the leap from animal models to human results is not always clean. The ethical issues surrounding animal testing are one thing, but the accuracy of these tests is another. Human bodies can react quite differently from animals to the same compound. Enter bioprinted human tissues. By testing drugs on human-like tissue without involving human subjects, companies can gather more precise safety data. This would reduce the potential for adverse side effects when drugs finally reach clinical trials.
Never-Before-Possible Treatments
3D bioprinting isn’t just about improving what we already do—it’s about making the impossible, possible. We’re talking about regenerative medicine that could one day restore lost limbs and teeth, or bioprinting custom skin grafts for burn victims. Researchers are even considering how bioprinting could be used to create hybrid tissues—structures that mix biological and synthetic components to enhance functionality.
Consider a printed cornea that not only restores sight but includes built-in UV protection, or a tissue replacement with embedded nanosensors to detect cancer early. These treatments were unimaginable a decade ago, but bioprinting is bringing them into focus. In a future where our own cells can be used to print complex tissues, we could see breakthroughs like spinal cord repairs that restore mobility or printed cartilage that halts arthritis in its tracks.
Challenges & Limitations of 3D Bioprinting
Printing a piece of plastic is hard enough. Printing something living—something that not just looks like an organ but functions like one—is orders of magnitude harder.
The Vascularization Problem
The first and perhaps most obvious challenge is technological. Vascularization, or building blood vessels into the tissues, is one of the greatest technical hurdles. Think of it like trying to construct an elaborate subway system beneath a city made of jelly. Without the intricate, spiderweb-like network of blood vessels, cells can’t get oxygen or nutrients, and tissue dies—essentially turning an ambitious living project into a failed science experiment.
Researchers are constantly working on solving this bottleneck. For instance, they are developing innovative methods like using 3D-printed sacrificial structures that dissolve to leave behind vascular channels, or engineering pre-vascularized tissues that contain cells prearranged to form vessels. Another emerging approach involves microfluidic bioprinting, which allows for the direct creation of tiny, perfusable channels that mimic blood vessels.
The Cost of 3D Bioprinting
Economic factors also play a significant role. A bioprinter can cost anywhere from $100,000 to over $500,000, depending on the sophistication and capabilities of the machine. For example, extrusion-based bioprinters typically cost between $10,000 to $200,000. More advanced laser-assisted bioprinters can exceed half a million dollars.
The materials themselves are also expensive. Stem cells can cost around $5,000 per vial. Growth factors necessary for cell differentiation can range from $2,000 to $10,000 per milligram. And hydrogels, which provide the necessary scaffolding, can cost between $500 to $2,000 per liter. On top of these, there are costs related to cell culture media, which can add hundreds of dollars per batch. If you’re not keeping track of all that, you don’t need to—it’s a lot. Scaling this technology for mass production is a mountain yet to be climbed.
Regulatory and Ethical
Regulatory and ethical concerns also cast a shadow over the bioprinting landscape. Are we comfortable with printing human tissue? What about printing an entire human? There’s an almost Frankenstein-like quality to some of these questions, and the ethical debate has yet to catch up to the technology itself. Regulatory bottlenecks add another layer of complexity.
Currently, the process of getting bioprinted tissues approved for medical use is a maze of regulatory approvals. Agencies like the FDA in the United States are still developing for the unique risks and challenges of bioprinting. What counts as ‘safe’ when you’re creating living tissues? Should the regulations resemble those for medical devices, drugs, or something entirely new? These uncertainties create a significant bottleneck for commercialization.
Beyond safety, there are regulatory questions about ownership and intellectual property. If a hospital prints a kidney using a patient’s own cells, who owns that kidney? Does the patient? The hospital? Or perhaps even the biotech company that designed the bioink? That sounds almost farcical to ask, but they’re important to address proactively. Ethical concerns like these blur the lines between innovation and exploitation, and the lack of clear legal frameworks makes some investors and companies wary.
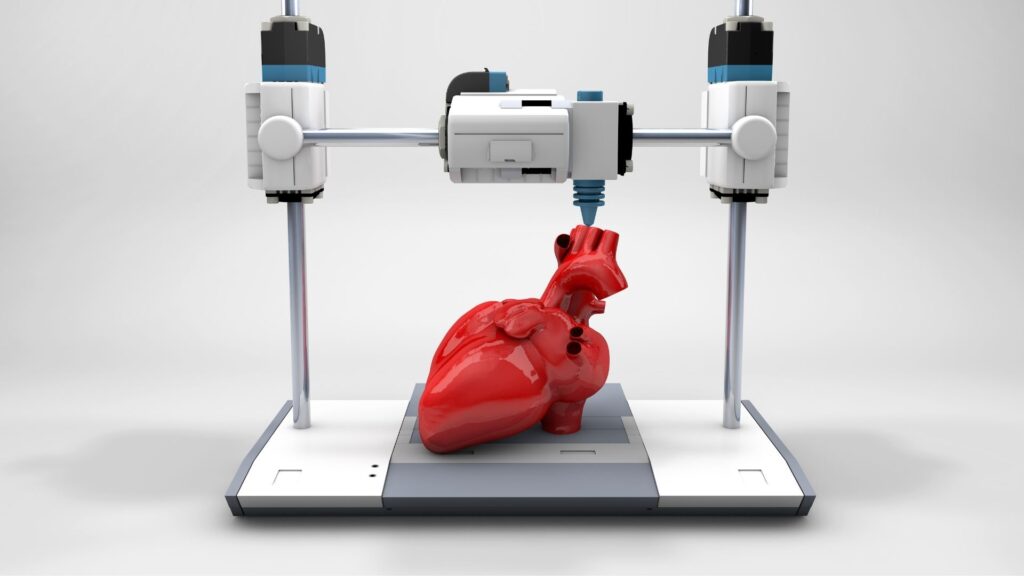
Applications of 3D Bioprinting
The applications of bioprinting are profound, but early adopters will be heavily concentrated in a few key industries.
3D Bioprinting in Healthcare
The most obvious and impactful use of bioprinting is in healthcare and biotech. From regenerative medicine to organ transplants, bioprinting promises to change lives. Right now, printed tissues are being used as skin grafts for burn victims, as cartilage replacements, and as patches for damaged hearts. Surgeons dream of the day when they will be able to print organs—not on a nationwide scale, but on a hospital-by-hospital basis.
For instance, researchers at Wake Forest Institute for Regenerative Medicine have already created bioprinted ear, bone, and muscle structures. When implanted in animals, they successfully matured into functional tissue. In the Netherlands, Utrecht University is working on bioprinting kidney tissues with functioning tubules, aiming to address the critical shortage of kidneys available for transplantation.
3D Bioprinting in Pharma & Biotech
Pharmaceutical companies are also using bioprinting to test drugs on human tissue models. It’s safer, quicker, and ultimately, more accurate. Organovo, for example, has developed bioprinted liver tissue that pharmaceutical companies are using to test drug toxicity. AstraZeneca has also adopted bioprinting technology to create lung and liver tissues to predict how patients might react to new treatments.
One of the most promising frontiers for 3D bioprinting is disease modeling. Researchers are beginning to use bioprinted tissues to recreate disease environments in the lab. They essentially build ‘mini-organs’ that are affected by specific diseases like cancer or fibrosis. These bioprinted models allow scientists to study the progression of diseases in ways that are impossible in live patients. For instance, they can print cancerous liver tissues to see how tumors develop and react to different drugs.
3D Bioprinting Meat in the Food Industry
It’s not just human tissue that’s being printed—scientists are also working on printing meat. The goal is simple: replicate the taste and texture of a steak without involving a cow. Companies like Aleph Farms and Memphis Meats (now known as Upside Foods) are already producing prototypes of lab-grown meat that look, cook, and taste like the real thing. Aleph Farms, in particular, has successfully created lab-grown ribeye steak using bioprinting technology, complete with muscle fibers and fat marbling.
Meanwhile, Mosa Meat, a Dutch company, has developed bioprinted beef burgers that have been taste-tested and found to be nearly indistinguishable from conventionally raised beef. For anyone concerned with animal rights, environmental degradation, or simply with the ethics of eating animals, this development is huge. The potential impact on the environment is also a big factor. Lab-grown meat uses significantly less water, land, and emits fewer greenhouse gasses compared to traditional livestock farming.
3D Bioprinting in Space
NASA and other space agencies have a keen interest in bioprinting, and for good reason. Imagine you’re on a multi-year mission to Mars and someone on the crew needs an organ transplant or suffers a severe burn. You can’t exactly call for a medical evacuation. Having a bioprinter on board could be a game changer, offering a way to create tissues or even entire organs on demand.
In 2019, Russian cosmonauts on the International Space Station used a 3D bioprinter to print a small amount of human cartilage and a rodent thyroid gland, demonstrating that bioprinting can work in microgravity. Even more mundane applications—like printing food or tissues for research—could be instrumental for long-term missions.
Redwire Space has also been working with Techshot to develop BioFabrication Facility (BFF), a bioprinter designed to function in microgravity, aiming to produce human tissues for research and therapeutic purposes during space missions. This capability could be essential for maintaining crew health on long-term space explorations, where medical emergencies must be handled on-site.
The Road Ahead
Over the next three years, one of the biggest areas to watch in the bioprinting space is regulatory progress. The FDA and similar agencies around the world are beginning to develop guidelines for bioprinted products. These policies will need to cover skin grafts, tissue patches, and more complex organ systems. Companies like Organovo and CELLINK are working closely with these agencies to push bioprinted products through the pipeline.
A breakthrough here could massively accelerate the adoption of bioprinted tissues. Industry insiders expect that the first commercial use for bioprinted tissues might not be entire organs, but smaller, less complex structures like cartilage or corneal implants. Regulatory clearance for these “gateway” applications would be a critical first step toward broader, more ambitious uses.
Another key trend is the strategic partnerships between bioprinting companies and big pharma. In the coming years, more bioprinted tissues will be integrated into drug discovery pipelines. Companies like AstraZeneca have already started using bioprinted liver and lung tissues in their testing. If these partnerships succeed, we could see a drastic reduction in the costs and timescales associated with bringing new drugs to market.
3D bioprinting is one of those fields where it feels like science fiction is becoming reality, inch by inch, layer by layer. It’s already starting to make headlines, attract investment, and open up doors we hadn’t even thought to knock on. Sure, there are challenges—technical, ethical, and financial—but each new advance makes the vision a little more tangible. And while we’re not yet at the point where doctors can “press print” on a new liver, we’re well on the way.